Neuroimaging Reveals How LSD Affects the Brain
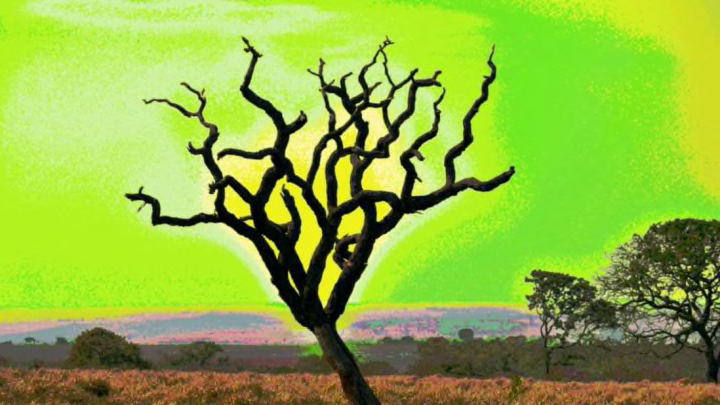
Since its invention some 80 years ago, LSD has been considered one of the most powerful psychedelic drugs, with a mysteriously high ability to expand the conscious experience beyond the confines of the body. But what does this enigmatic drug actually do inside the brain? Thanks to the first-ever study of LSD with modern brain-imaging techniques, we now have a glimpse of the psychedelic in action.
Robin Carhart-Harris and David Nutt of Imperial College London and their colleagues looked at changes in brain activity patterns during the hallucinatory and consciousness-altering effects of LSD (lysergic acid diethylamide). They found a pattern of communication across the brain that could explain the drug's profound sensory and mind-altering effects. They published their findings today in the journal Proceedings of the National Academy of Sciences.
In the study, divided into two sessions that took place on two days, 20 participants received an IV infusion of either LSD or a salt-water placebo. They then lay in a brain scanner with their eyes shut. During each roughly four-hour session, the participants underwent neuroimaging with multiple techniques, including functional magnetic resonance imaging (fMRI) and magnetoencephalography (MEG).
During the session when subjects had LSD in their system, much more of the brain was involved in visual processing compared with the placebo session. Moreover, the visual cortex, the part of the brain involved in processing visual information, showed greater synchronous activity with many areas of the brain. The greater this connectivity, the higher the participants’ reporting of complex visual hallucinations.
Carhart-Harris et al. in PNAS
“What was really intriguing was the magnitude of this expanded visual processing, which was correlated with people's ratings of complex visual hallucinations—the kind of dreamlike visions they describe with psychedelics, involving landscapes and people,” Carhart-Harris told mental_floss.
Meanwhile, as expected, people experienced altered consciousness states as well. One such experience involved a disintegration of the sense of self, or what researchers call ego-dissolution; it was linked with decreased connectivity between two brain regions, the parahippocampus and retrosplenial cortex, suggesting this connection is linked to a sense of self. This was part of a general disruption in the default mode network—a network of brain regions that normally shows a robust pattern of connectivity as people are awake and resting, thinking, remembering the past, and planning for the future.
“The findings are quite consistent with previous findings on psychedelics,” Carhart-Harris said. “We are now getting more confidence in understanding what underlies subjective experience produced by psychedelics.”
"FUNDAMENTAL CHANGES IN CONSCIOUSNESS"
Putting together these results with brain imaging findings of other psychedelics points to some general principles, Carhart-Harris said. It seems that LSD breaks the boundaries between well-established brain networks, giving rise to a different, more flexible form of communication among them.
During the development of the brain, neural networks become specialized in the tasks they perform. As these networks become more and more distinct from each other, the communication between them becomes less flexible. “With LSD, these networks in the brain begin to lose their integrity. You see a desegregation of brain systems, where networks start to blend with each other. On the whole, the brain becomes more globally connected, operating in a more flexible way,” Carhart-Harris said. “And this seems to map with some of the fundamental changes in consciousness that you see with LSD.”
"This powerhouse of a study employs a number of cutting-edge human neuroscience techniques to examine the effects of LSD on brain activity,” said Gaurav Patel, a psychiatrist at the New York State Psychiatric Institute at Columbia University Medical Center, who was not involved with the study. The use of multiple techniques in single individuals to study the changes in brain activity helps free the researchers from potential confounds in any one technique, Patel said. “Moreover, the findings were relatively specific, and had high correlations with behavioral measures,” Patel told mental_floss.
ILLUMINATING LSD FOR SCIENTISTS AND NON-SCIENTISTS ALIKE
For an old drug with such intense effects on the brain, very little is known about LSD. After it was first synthesized by Albert Hofmann in 1938, LSD found its way into psychiatric settings and was in use throughout the 1950s and '60s. The drug also presented an intriguing opportunity for research. Between 1953 and 1973, the U.S. government alone funded more than 100 studies of LSD. But the drug was ultimately banned under the United Nations Convention on Psychotropic Substances, and fell off the radar of researchers due to political and social stigma.
But in recent years, LSD and other psychedelics have gained a renewed interest as potentially untapped resources useful for mental health treatment or studying consciousness. This interest is shared by scientists and nonscientists alike. For the present study, the researchers asked the public to cover the remainder of the cost for finishing the experiment in a crowdfunding campaign last year, ultimately raising £53,390 (about $80,000)—more than double their original goal (the study was also funded by the Beckley Foundation).
“The response was amazing,” said Carhart-Harris, who sees this as evidence of a genuine intellectual interest among the public for understanding the curious effects of the drug.
Carhart-Harris and colleagues previously studied psilocybin, the active compound in psychoactive mushrooms. They found psilocybin allowed for bypassing the brain's normal control, lifting the typical limits on our perception—an idea reminiscent of what Aldous Huxley suggested in his 1954 book on psychedelics, The Doors of Perception.
The new findings on LSD, too, suggested the drug disrupts the normal pattern of activity in important brain networks, allowing the brain to operate in a more flexible, fluid way, Carhart-Harris said.
The researchers suggest this modification of normal brain communication underlies ego-dissolution. There isn’t a clear definition of this phenomenon yet, but Carhart-Harris describes it as a feeling of becoming less sure of the self, identity, and personality. “You begin to see your ‘self’ more as something objective as opposed to subjective," he said. "This often is accompanied by certain insights about oneself, one's background and relationships with others and with the world in general. And actually it often goes hand-in-hand with feelings of a spiritual and mystical nature.”
THE POTENTIAL FOR UNDERSTANDING—AND TREATING—DISORDERS
In another article published online in the May issue of Psychological Medicine, the team detailed the findings on psychological effects of LSD. One paradoxical effect of the drug, the team said, was that it includes psychosis-like symptoms when it’s taken—yet seems to improve psychological well-being afterward. It is possible that LSD increases cognitive flexibility and leaves a residue of “loosened cognition” that leads to improved psychological well-being, the researchers said.
A few other studies, too, have explored the possible positive effects of LSD or other psychedelics on mental health. A 2014 study with 12 people with life-threatening diseases, for instance, found LSD useful for easing anxiety. And when researchers followed up with nine of the people a year later, they found the effects to be long-lasting.
Studying how psychedelics affect the brain can reveal new insights about how the brain works, in both health and disease.
“In psychiatric research, we struggle with understanding how the brains of individuals may or may not be different from what they could have been if healthy,” Patel said. “Here, we get to see how psychiatric-like symptoms correlate with circuit-level changes in brain activity. It is rare to see a study of this nature performed so rigorously, and to have found such clean results.”