Walk This Way: The History of the Moving Sidewalk
By Matt Soniak
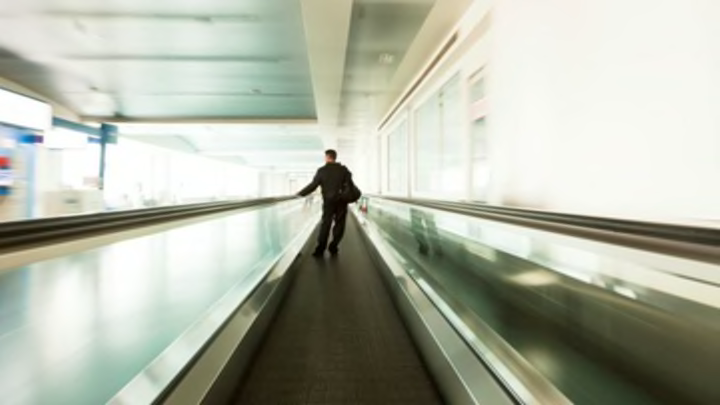
Authors like H.G. Wells, Isaac Asimov, and Robert Heinlein have long envisioned a future where the automobile gives way to massive, high-speed moving walkways. Some of them merely zoom commuters around cities, while others, like Heinlein’s "mechanized roads,” could take people from Cleveland all the way to Cincinnati.
The moving sidewalk is familiar to travelers in the real world, too, but smaller and limited to controlled environments like airports and train stations. They lack the grandeur and the game-changing status that futurists once envisioned, but that isn’t to say that people haven’t tried for larger, longer, faster moving sidewalks. Inventors had very real plans for the moving sidewalk that rivaled anything Wells dreamed up, but were undone by technical limitations and wary politicians and riders that science fiction authors could simply write their way around.
A Walking Tour of Moving Sidewalks
The history of real-world moving sidewalks goes back to a New Jersey inventor/wine merchant named Alfred Speer, who received the first patent for one in 1871. The first one operated in the U.S. was built for the 1893 World's Fair in Chicago. Operated by the Columbian Movable Sidewalk Company, which charged 5 cents for a ride, it ran almost the entire length of the 3,500-foot pier that many guests arrived at after taking a scenic steamship trip from downtown to the fairgrounds. Riders could stand or walk on the first platform, which traveled at about two miles per hour, or step up onto a second parallel platform, which ran at four miles per hour and had benches. Running at full capacity, the walkway could ferry 31,680 passengers per hour. Its life was short, though, and it was destroyed by a fire the following year.
The wooden moving pavement ('Trottoir Roulant') at the Exposition Universal in Paris, 1900 /
In the early years of the next century, Speer and Max Schmidt, who designed a moving walkway for the 1900 Exposition Universal in Paris, both proposed their own versions of the moving sidewalk in Manhattan to relieve some of the foot traffic on New York City’s crowded streets. Speer’s plan called for an elevated system of three parallel walkways running along Broadway that would move passengers at up to 19 mph. Speer’s system had one stationary platform for boarding and two moving ones where riders could either stand, walk or even have a seat in one of a few enclosed “parlor cars” that had drawing rooms for ladies, and space for men to sit and smoke. Despite building a working model and finding support in the city government and state legislature, Speer’s project was repeatedly killed by the governor.
Schmidt’s vision for a Brooklyn Bridge moving walkway consisted of a loop system with four platforms, one for boarding and three others that moved at increasing speeds, the fastest of which ran at 10 mph. Schmidt planned for the system to run constantly, so passengers wouldn’t have to wait to board and no momentum would be lost on stopping and starting the platforms. Schmidt and the individuals and groups who proposed similar systems in Atlanta, Boston, Los Angeles, Detroit, and Washington, D.C., all eventually saw their plans crumble under their own novelty. Maintenance and breakdown concerns, the question of what passengers were supposed to do in the rain or snow, and the familiarity and reliability of buses and subway trains all helped doom the urban moving sidewalk.
Let’s Try This Again
A half-century later, the moving sidewalk reared its head again when smaller-scale versions showed up in sprawling airports and train stations. They’re hardly the stuff of Wells and Schmidt, and are usually just a single platform moving slowly from Point A to Point B just a few hundred yards away.
The first of these simpler sidewalks got moving in May 1954 at the Hudson and Manhattan Railroad’s Erie station in Jersey City, NJ. Built by the Goodyear Tire and Rubber Co., the “Speedwalk’s” 5½-ft wide platform ran 277 feet up an incline used to exit the station, at a top speed of 1.5 mph. It was a relief to many riders used walking up the exit hall, which had earned the nickname “Cardiac Alley.”
While the Speedwalk might have prevented a few injuries, the first moving sidewalk installed at an airport - at Love Field in Dallas in 1958 - infamously caused several. One person was even killed. Early in the sidewalk’s operation, several people got clothing or a foot stuck where the conveyor met solid ground and disappeared into the floor to loop back. A dog suffered a broken leg. A seven-year-old boy got his t-shirt and hand sucked in and lost most of the skin on his fingers. As the boy’s mother tried to free him, her clothing got caught too, and her skirt and slip were pulled clean off. She continued to struggle with her son in nothing but a leather coat until the machine was turned off.
Two years later, an accident resulted in death. On New Year’s Day in 1960, a two-year-old girl, fascinated by the moving sidewalk, broke away from her mother and waddled over for a closer look. Her coat sleeve got caught at the edge, and her left hand, wrist and forearm were pulled below the floor. A police officer rushed to cut off her clothes to release her. He later told newspapers that her coat was pulled so tight around her chest that he couldn’t even get his knife underneath it.
Not So Fast
Designs and safety measures for the moving sidewalk improved, and its use spread to most airports over the next few decades. Some engineers even took another stab at larger, faster versions. Prototype high-speed walkways have been tried out in Paris metro stations in the 1980s and the early 2000s, but both systems were shut down due to mechanical complexity, unreliability, and passenger accidents.
While the idea of quickly floating over the Brooklyn Bridge or across Ohio on a moving walkway is exciting, there seems to be a practical limit to how fast a person can travel on a moving platform without losing their balance and toppling over. Fast, car-less travel over great distances is maybe best left to airplanes and high-speed rail lines. For the shorter moving sidewalks we have now, we don’t necessarily need speed and all the mechanical and safety problems that go with it.
The airport moving sidewalks often slow us down, versus walking normally, because people stand around or block the platform with their bags. The future of the people mover, perhaps, isn’t in a mechanical road that takes us from one town to another, but just an airport moving walkway that isn't treated like a leisure cruise. As Jerry Seinfeld used to say, "It's not a ride!"